Introduction of Bohr Effect and Root Effect
The Bohr Effect and Root Effect are two physiological phenomena related to hemoglobin’s binding and release of oxygen in vertebrates. Christian Bohr’s discovery, the Bohr Effect, describes how hemoglobin’s affinity for oxygen varies with changes in pH.
Its regions with lower pH environments like actively metabolicizing tissues often require lower affinity hemoglobin which releases oxygen more freely for tissue needs than regions with higher pH environments such as lung tissue where it binds it more securely.
On the other hand, the Root Effect, named for R. W. Root, can be observed primarily in fish and some invertebrates. It represents a mechanism by which hemoglobin releases oxygen even under high oxygen tension conditions as seen through their gills when oxygen tension levels increase dramatically.
Compared with the Bohr Effect, the Root Effect relies less heavily on pH changes for its release capacity of oxygen similar to its name indicates. Both effects play an essential role in optimizing oxygen transport between organisms and demonstrate how nature has developed strategies for providing efficient oxygen delivery to tissues under diverse physiological circumstances.
Definition of Bohr Effect
The Bohr effect refers to the change of the oxygen dissociation curve triggered by variations in the level of carbon dioxide, or in the pH of the surrounding environment. The Bohr effect was first reported by the Danish biochemist Christian Bohr in 1904.
The reaction of carbon dioxide with water creates carbonic acid. Thus, the increase in CO2 could cause a drop in the blood pH. It also causes hemoglobin protein to let out oxygen. The reduction in carbon dioxide could trigger an elevation in pH, which may result in hemoglobin picking the oxygen up.
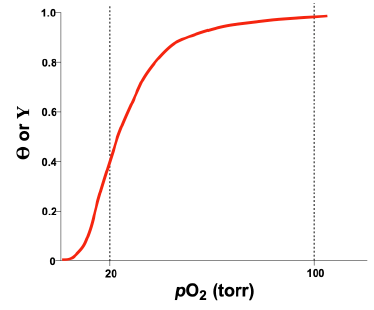
The Bohr effect improves the efficiency of the oxygen transport process through blood. In the wake of the hemoglobin binding to oxygen within the lungs because of the large oxygen levels in the lungs.
The Bohr effect could facilitate the release of oxygen in tissues, particularly the ones that require the greatest amount of oxygen. As the metabolism rate of the tissue increases and carbon dioxide is formed, protons and bicarbonate also rise.
The process usually takes a while. The carbonic anhydrase enzyme significantly speeds up the transformation of protons and bicarbonate. This, in turn, results in the pH of blood to drop. The process also aids in the dissociation of hemoglobin with oxygen and allows tissues around to get enough oxygen to satisfy their needs.
Mechanism at the Molecular Level
The Bohr Effect operates at a molecular level by altering hemoglobin’s structure and properties; this article offers more detail.
Here’s an in-depth description of this phenomenon:
- Hemoglobin Structure: Hemoglobin is a complex protein composed of four subunits, each containing an iron-containing heme group that binds oxygen atoms. Each iron heme group can bind one oxygen molecule.
- pH-Dependent Protonation: The Bohr Effect is driven primarily by changes in pH (acidity). When metabolically active tissues such as muscles during exercise become metabolically active, carbon dioxide production from cellular respiration rises resulting in CO2 being combined with water (H2O) via carbonic anhydrase enzyme to form carbonic acid (H2CO3) which then releases protons (H+) back into surrounding tissue.
- Proton Binding to Hemoglobin: Increased concentrations of protons (H+) in acidic environments of metabolic tissues lead to protonation of specific amino acid residues on hemoglobin, specifically histidine residues that accept protons as protons are released.
- Conformational Changes: When protons bind to histidine residues in hemoglobin molecules, this leads to conformational changes that reduce its affinity for oxygen and cause it to release it more readily from storage pools.
- Enhance Oxygen Release: Due to reduced oxygen affinity, hemoglobin can release oxygen more readily in tissues with lower pH (higher acidity). This benefit is particularly important since metabolically active tissues require additional oxygen supply; Bohr’s Effect ensures it reaches where it’s most needed.
- Oxygen-Hemoglobin Dissociation Curve: The Bohr Effect is typically visualized as a rightward shift of the oxygen-hemoglobin dissociation curve, signifying that hemoglobin has less affinity for oxygen in acidic environments and thus facilitates its release more easily.
Definition of Root Effect
Root effect is a sign that an increase in carbon dioxide or proton concentration decreases hemoglobin’s capacity and affinity for oxygen. The result is a natural phenomenon within the hemoglobin of fish.
The hemoglobin that exhibits the Root effect is characterized by a loss of cooperativity when pH is low. This causes the Hb-O2 dissociation curve to shift upwards, not only to the left. Hemoglobin exhibits the Root effect. It is not fully oxygenated even with oxygen tensions of that exceed 20 kPa.
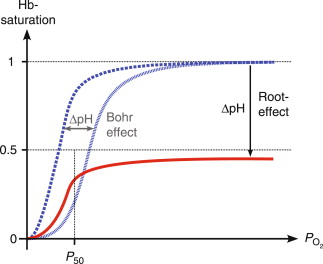
Additionally, this feature permits the bladder to move against an oxygen gradient that is high which is evident in the choroid rate in which the blood vessels could transport oxygen into the retina. If there isn’t a Root Effect, the retia process results in the dispersal of oxygen that is directly coming from the bloodstream to the blood vessels of the veins.
The result is that these systems become not as effective in the amount of oxygen. There is a hypothesis that loss affinity can be beneficial by providing greater oxygen levels to the red muscle during acidotic stress.
Mechanism and Unique Hemoglobins
Here is an explanation of the mechanism and unique hemoglobins associated with Root Effect:
Root Effect Mechanism:
- Structure Variations: Hemoglobin variants involved in the Root Effect have distinct structural variations compared to typical hemoglobin, and this difference impacts their oxygen-binding capabilities.
- Low Oxygen Affinity: Hemoglobin variants associated with the Root Effect stand out by having an inherently lower oxygen affinity; these variants readily release oxygen even when oxygen tension in their environment or blood is relatively high.
- Functional Implications: The Root Effect is often activated by specific physiological circumstances, such as rapid swimming in fish. As tissues experience increased oxygen demand during these activities, unique hemoglobin variants respond by efficiently releasing oxygen so it reaches muscles or tissues engaging in high metabolic activity.
Unique Hemoglobins for Root Effect:
- Hemoglobin Variants: Organisms that display the Root Effect often possess hemoglobin variants or isoforms tailored specifically for oxygen transport needs in their ecologies, evolving to meet these challenges and adapt accordingly.
- Evolutionary Adaptation: The presence of these unique hemoglobin variants reflects an evolutionary response to specific environmental conditions, for instance fish living in oxygen-poor aquatic environments have developed Root Effect hemoglobins to thrive under conditions where other forms of hemoglobin might not work as efficiently.
- Environmental Factors: The Root Effect is especially useful in environments with fluctuating oxygen availability, such as deep waters or fast-flowing streams where oxygen tension drops dramatically. Fish equipped with Root Effect hemoglobins can efficiently extract and deliver oxygen even under such circumstances, providing benefits even during low oxygen tension conditions such as deep diving or swift stream travel.
Key Difference Between Bohr Effect and Root Effect
The Bohr effect as well as Root effect are significant effects. The major distinction between the Bohr effect as well as Root result is that with the Bohr effect it is only the affinity for oxygen is decreased, while in the Root effect, the affinity as well as the carrying capacity of oxygen is decreased.
Here’s a simplified comparison chart between the Bohr Effect and Root Effect:
Aspect | Bohr Effect | Root Effect |
---|---|---|
Discoverer | Christian Bohr (20th century) | R. W. Root (20th century) |
Mechanism | pH-dependent oxygen binding | pH-independent, enhanced oxygen release |
Organisms | Vertebrates, including humans | Fish and some invertebrates |
Oxygen Affinity | Reduced affinity in acidic environments | Enhanced oxygen unloading in specific situations |
Tissue Activity | Optimizes oxygen delivery in metabolizing tissues | Increases oxygen release during activities |
Hemoglobin Function | Balances oxygen loading and unloading | Promotes efficient oxygen unloading |
Oxygen Tension | Influenced by pH changes | Less sensitive to pH changes |
Evolutionary Origin | Likely an ancient evolutionary mechanism | Evolved in specific lineages |
Overall Role | Facilitates oxygen transport to tissues | Enhances oxygen unloading when needed |
Medical Significance | Relevant in respiratory and metabolic disorders | Less medically significant |
Comparative Rarity | Common in vertebrates | Primarily observed in fish and select invertebrates |
Explaining the Molecular Mechanisms of the Bohr and Root Effects.
The Bohr Effect depends on pH changes to regulate hemoglobin’s oxygen-binding affinity, as detailed here.
Here is more of an in-depth explanation of its molecular mechanisms:
- Hemoglobin Structure: Hemoglobin is a four-subunit protein composed of iron-containing heme groups that each bind one oxygen molecule.
- pH and Hemoglobin Structure: Environmental pH can have an impactful impact on certain amino acid residues within hemoglobin. Histidine residues within hemoglobin, for instance, can either become protonated (H+) or deprotonated (H-) depending on pH levels in their surroundings.
- Effect of pH on Hemoglobin: When placed in an acidic environment with lower pH values, such as those encountered during metabolic activity in tissue cells, more protons (H+) become available and attach themselves to specific histidine residues on hemoglobin, inducing conformational changes within its protein.
- Conformational Changes: Protons binding with hemoglobin cause it to undergo conformational changes that decrease its affinity for oxygen, making it more likely that hemoglobin releases oxygen into tissues with lower pH (higher acidity) where oxygen is required for metabolism.
- Effect on Oxygen Binding Curve: The Bohr Effect shifts the oxygen-hemoglobin dissociation curve right, signifying lower affinity in acidic conditions and encouraging oxygen release.
- Molecular Mechanism of the Root Effect: The Root Effect, observed most frequently among fish and select invertebrates, is a molecular process that increases oxygen unloading under certain circumstances. Compared with its counterpart, the Bohr Effect, the Root Effect relies less heavily on pH changes for its effectiveness while employing multiple molecular mechanisms:
- Specialized Hemoglobin Variants: Organisms that exhibit the Root Effect typically possess customized hemoglobin variants or isoforms designed specifically to meet their oxygen transport requirements.
- Unique Hemoglobin Structure: Hemoglobin variants involved in the Root Effect may feature unique subunit structures compared to traditional hemoglobin.
- Effect on Oxygen Affinity: Specialized hemoglobin variants have a reduced oxygen affinity, enabling them to release more readily even under high oxygen tension conditions.
- Specific Triggers: The Root Effect may be initiated by specific physiological conditions, such as high metabolic demand, physical activity or changes to environmental oxygen levels.
- Enhance Oxygen Unloading: When activated, the Root Effect allows for increased oxygen unloading from hemoglobin, providing tissues with adequate supplies of oxygen even during times of increased demand.
The Bohr and Root Effects in Oxygen Transport.
Bohr Effect on Oxygen Transport:
- pH Sensitivity: To regulate oxygen transport, the Bohr Effect primarily relies on changes to pH (acidity). Tissues with high metabolic activity such as muscles produce carbon dioxide as an exhaust gas which combines with water to form carbonic acid (H2CO3) which then lowers their pH level and makes their environment more acidic.
- Hemoglobin Response: Hemoglobin, the oxygen-carrying protein in red blood cells, is sensitive to changes in pH. When exposed to acidic environments, hemoglobin undergoes a conformational change as protons (H+ ions) bind with amino acid residues of its protein structure resulting in binding protons bind specific amino acid residues binding protons which in turn reduce its affinity for oxygen.
- Enhancing Oxygen Release: With decreased oxygen affinity, hemoglobin releases oxygen more readily in tissues with lower pH. This benefit is especially significant as metabolizing tissues have higher needs for oxygen; with the Bohr Effect guaranteeing its delivery precisely where needed.
- Change in Oxygen-Hemoglobin Dissociation Curve: The Bohr Effect causes a shift of the oxygen-hemoglobin dissociation curve to the right, signifying lower affinity of oxygen for tissues in acidic environments and thus providing for efficient release of oxygen to tissues.
Root Effect of Oxygen Transport:
- Specialized Hemoglobin Variants: The Root Effect is observed in certain fish and invertebrates, and involves specialized hemoglobin variants or isoforms with distinct structural properties when compared with typical hemoglobin.
- Hemoglobin Variants involved: The Root Effect has lower oxygen affinity, meaning they can readily release oxygen even when oxygen tension in their environment is relatively high.
- Specific Conditions Trigger the Root Effect: The Root Effect can often be brought on by certain physiological conditions, such as high metabolic demands during vigorous swimming or exposure to low oxygen levels in aquatic environments.
- Enhance Oxygen Unloading: When activated, the Root Effect allows for increased oxygen release from hemoglobin to ensure tissues receive sufficient amounts of oxygen during times of increased demand.
What are the implications of the Bohr and Root Effects for respiratory physiology?
Bohr and Root Effects play an instrumental role in respiratory physiology as they facilitate oxygen transport and allow organisms to adapt to diverse environmental and physiological conditions.
Bohr Effect:
- Tissue Oxygen Delivery: The Bohr Effect ensures oxygen reaches tissues efficiently. Specifically, muscles engaged in exercise produce carbon dioxide which leads to decreased acidity (lower pH). This causes hemoglobin release more readily thus meeting increased demand.
- Lung Oxygen Uptake: When pH levels in the lungs are more alkaline (less acidic), hemoglobin’s affinity for oxygen increases; thus enabling it to pick up oxygen efficiently from abundant levels in the lungs using the Bohr Effect.
- Respiratory Disorders: Understanding the Bohr Effect is paramount when treating respiratory conditions like chronic obstructive pulmonary disease (COPD) and metabolic acidosis, where oxygen uptake and delivery may become problematic. Chronic Obstructive Pulmonary Disease (COPD), for instance, or metabolic acidosis can impair this vital process and impede oxygen uptake and delivery to tissues.
Root Effect:
- Root Effect in Fish: With fish being one of the highest consumers of oxygen, the Root Effect becomes especially relevant during activities like fast swimming that necessitate the release of oxygen from specialized hemoglobin variants. This release process is necessary in order to meet oxygen demands effectively.
- Adaptation to Hypoxic Environments: Fish living in oxygen-deficient aquatic environments benefit greatly from the Root Effect, as it enables them to extract oxygen efficiently even at relatively low concentrations in water.
- Environmental Adaptation: The Root Effect is an adaptation that allows fish to thrive under fluctuating environmental conditions by matching oxygen delivery with metabolic needs, even with fluctuating oxygen levels.
What is the evolutionary significance of the Bohr and Root Effects in different organisms?
Bohr and Root Effects hold enormous evolutionary significance across organisms. For vertebrates like us, such as humans, it probably represents an ancient adaptation that allowed early vertebrates to efficiently transport oxygen during metabolic activity – giving these organisms an edge in diverse environments.
Conversely, the Root Effect seen primarily among fish and invertebrates is an adaptation that developed specifically in response to specific environmental challenges. It enables these organisms to survive oxygen-poor aquatic habitats by increasing oxygen release during activities like rapid swimming.
These effects demonstrate the incredible adaptability and plasticity of hemoglobin. It has developed different mechanisms to adapt to meet different organisms’ oxygen transportation requirements – reflecting its significance as an oxygen carrier throughout evolution.
What clinical insights do the Bohr and Root Effects provide into oxygen therapy?
Bohr Effect in Oxygen Therapy:
- pH Sensitivity: Recognizing the Bohr Effect’s dependence on pH levels is essential in tailoring oxygen therapy effectively. If patients experience acidic environments during exercise or respiratory distress, for instance, then oxygen delivery must be adjusted accordingly in order to accommodate its influence.
- Customized Oxygen Delivery: Healthcare providers can leverage the Bohr Effect to personalize oxygen therapy regimens. By monitoring tissue acidification levels and adapting flow rates or concentrations as necessary to provide maximum oxygen unloading in metabolically active areas.
- Monitoring and Assessment: Healthcare professionals may find the Bohr Effect an invaluable indicator. Alterations in pH levels may indicate altered tissue metabolism or respiratory function, prompting timely adjustments to oxygen therapy plans.
Root Effect of Oxygen Therapy:
- Environmental Factors: For patients in environments with fluctuating oxygen levels or limited availability, insight from the Root Effect may prove especially helpful. It allows healthcare providers to better understand how different hemoglobin variants unload oxygen. This can be especially pertinent in high-altitude settings or travel to low-oxygen regions.
- Adaptive Strategies: Understanding the Root Effect enables healthcare professionals to appreciate how certain organisms, like fish, have evolved naturally to adapt to low oxygen conditions. This knowledge can provide healthcare providers with ideas for innovative oxygen therapy techniques for treating patients living in oxygen-poor environments.
- Patient-Specific Considerations: Some individuals may possess genetic variations of hemoglobin that exhibit Root Effect-like properties. Recognizing these variants can assist in tailoring oxygen therapy specifically to their unique needs and ensure efficient oxygen unloading.
Summary
The Bohr Effect and Root Effect are two distinct physiological mechanisms related to oxygen binding and release by hemoglobin in different organisms. Christian Bohr first identified this mechanism; it’s pH-dependent and widely observed among vertebrates such as humans. It regulates oxygen binding and release with reduced affinity in acidic environments to ensure efficient oxygen delivery to metabolizing tissues.
Root Effect, named after R. W. Root, can be found primarily in fish and certain invertebrates. It is pH-independent and works to facilitate oxygen unloading during activities or high oxygen conditions; unlike Bohr Effect which is more sensitive to pH changes. Root Effect evolved as part of specific lineages to optimize oxygen transport.
Bohr Effect has immense medical importance for conditions like respiratory and metabolic illnesses, while Root Effect may have less medical bearing. Both mechanisms illustrate nature’s diverse strategies for providing oxygen transport across different physiological contexts.