Introduction
Strong and Weak Electrolytes ionize or dissociate into ions when dissolved in a solvent, enabling the solution to conduct electricity.
Electrolytes are a central concept in chemistry, playing an essential role in many chemical reactions, biological processes, and everyday applications. Electrolytes are substances that, when dissolved in a liquid such as water, can conduct electricity due to free-moving ions present within their structure. Understanding their behavior and properties is integral for understanding complex chemical systems’ impact across diverse fields such as medicine, industry, and environmental sciences.
In this research paper, we explore the exciting world of electrolytes by exploring their distinction between strong and weak electrolytes. Both categories represent distinct classes of substances with specific characteristics, behaviors, and implications in chemical and physical processes; by exploring these distinctions between strong and weak electrolytes we aim to shed light on their roles and significance across a range of applications.
This investigation begins by outlining what electrolytes are and their significance in chemical reactions, before proceeding to introduce two broad categories of electrolytes: strong electrolytes that undergo near complete dissociation into ions upon dissolution in liquid, and weak electrolytes which only partially ionize in solvent. We will discuss key characteristics and representative examples of each type, in addition to conductivity and conductance measurements for each.
We will examine the factors affecting electrolyte strength such as substance type, concentration level, and temperature. By understanding these variables we can gain a better understanding of the dissociation process as well as the conductivity of electrolyte solutions.
This study also seeks to demonstrate the practical significance of understanding electrolyte strength. We will look into various applications, such as electrochemical processes, water treatment, and pH regulation where knowledge of strong and weak electrolytes plays an essential part. This understanding may have applications within medical and biological contexts providing insights into their behavior within biological systems.
As we delve deeper into strong and weak electrolytes, we hope to gain a better understanding of their place within chemistry and life beyond it. By uncovering their individual properties and behaviors, we hope to foster an appreciation for all the diversity, complexity, and contributions chemical systems make toward shaping our surroundings. So come join us as we unlock their mysteries!
Definition of Strong Electrolytes
Strong electrolytes are substances that fully dissociate or ionize into ions when dissolved in a solvent, typically water. That means they undergo near 100% dissociation into positively charged cations and negatively charged anions that are free to move around the solution freely and conduct electricity as part of its solution.
Strong electrolytes include strong acids, bases, and highly soluble ionic compounds. When added to water, these substances quickly decompose into their constituent ions resulting in an increase in electrical conductivity in the solution.
Key characteristics of strong electrolytes:
Dissociation: Strong electrolytes dissipate into their constituent ions when dissolved in a solvent.
Formation of Ions: Dissociation of strong electrolytes leads to the creation of positively charged cations and negatively charged anions, both positively charged.
Strong electrolytes: Include Hydrochloric acid (HCl), sulfuric acid (H2SO4) and nitric acid (HNO3) as Strong acids; sodium Hydroxide (NaOH) and potassium hydroxide (KOH) are Strong bases; while ionic compounds include Sodium chloride (NaCl) and Potassium nitrate (KNO3) are ionic Compounds.
Strong electrolytes play an integral role in many chemical reactions, electrochemical processes, water treatment procedures and biological systems due to their complete dissociation and high conductivity.
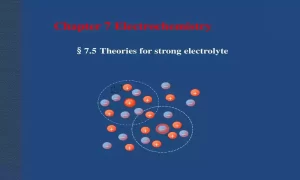
Definition of Weak Electrolytes
Weak electrolytes are substances that only partially dissociate into ions when dissolved in a solvent, such as water. Unlike their stronger counterparts, which usually dissociate nearly completely into their constituent ions when dispersed through the solution, weak electrolytes typically experience only limited dissociation into molecular and ionic forms in solution.
Key characteristics of weak electrolytes:
- Partial Dissociation: Electrolytes that dissolve only partially are known to partially dissociate in solvent solutions, meaning that most of their original molecules remain intact in molecular form.
- Equilibrium Between Ionic and Molecular Forms: When working with weak electrolytes, equilibrium exists between dissociated ions and undissociated molecules in solution, determined by chemical equilibrium principles as well as factors such as concentration and temperature. This equilibrium may change depending on several variables such as concentration or temperature fluctuations.
- Examples of weak electrolytes: include weak acids and bases as well as slightly soluble ionic compounds. When added to water, only some molecules dissociate into ions while most remain unchanged.
- Some examples of weak electrolytes: Include acetic acid (CH3COOH) as an acid, ammonia (NH3) as a base, and semi-soluble ionic compounds like lead chloride (PbCl2) and silver bromide (AgBr).
- As weak electrolytes only partially ionize: Their electrical conductivity is lower than that of strong electrolytes; nevertheless, they still play key roles in chemical reactions, pH regulation, biological processes, buffering solutions, and maintaining equilibrium within various systems.
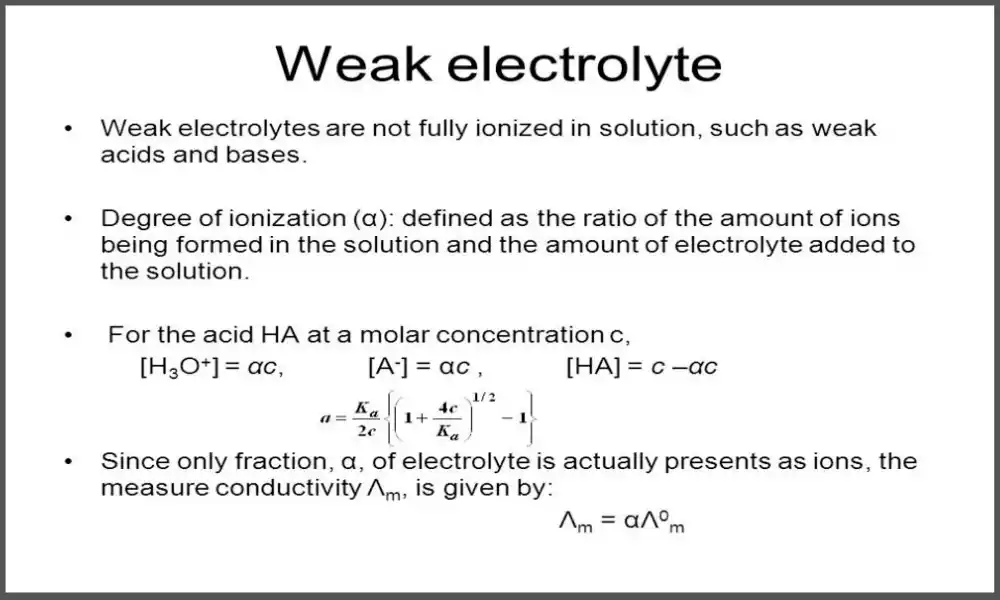
Comparison Table of Strong and Weak Electrolytes
Below is a comparison table highlighting the key differences between strong and weak electrolytes:
Feature | Strong Electrolytes | Weak Electrolytes |
---|---|---|
Dissociation | Almost complete dissociation into ions. | Partial dissociation into ions. |
Degree of Ionization | High ionization efficiency. | Low ionization efficiency. |
Conductivity | High electrical conductivity. | Low electrical conductivity. |
Examples | Strong acids (HCl, H2SO4, HNO3), | Weak acids (CH3COOH, H2CO3), |
Strong bases (NaOH, KOH), | Weak bases (NH3, C5H5N), | |
Highly soluble ionic compounds (NaCl, KNO3). | Slightly soluble ionic compounds (PbCl2, AgBr). | |
Equilibrium | Rapidly shifts towards ionization. | Establishes an equilibrium between ions and molecules. |
Conductance | High conductance due to abundant ions. | Low conductance due to limited ions. |
Electrical Applications | Widely used in various electrochemical processes, | Used in buffering solutions and maintaining |
such as batteries, fuel cells, and electroplating. | equilibrium in certain systems. | |
Biological Implications | Significantly impact pH and ionic balance in | Play a role in maintaining biological pH balance and |
biological systems. | ionic concentrations. |
Importance of Electrolytes in Chemical Reactions
Electrolytes play an essential role in chemical reactions due to their ability to conduct electricity when dissolved in solvents like water. Their importance lies in helping and driving various processes essential for various industries, biological systems and everyday applications.
Some key aspects of electrolytes’ role include:
- Facilitation of Ionic Reactions: Electrolytes play an essential role in reactions involving ionic compounds. As electrolytes dissolve into solvents, they dissociate into individual charged particles that facilitate reactions between these charged particles – for instance in electrolysis where this dissociation allows ions to migrate and participate in redox reactions.
- Enabling Redox Reactions: Electrolytes play an integral part in many redox (reduction-oxidation) reactions, in which one species undergoes oxidation (the loss of electrons), while another species experiences reduction (gaining electrons). Electrolytes facilitate these processes by helping transfer electrons between species allowing chemical energy to be transformed into electrical energy and vice versa.
- Acid-Base Reactions: Electrolytes, particularly strong acids and bases, play an integral part in acid-base reactions. Electrolytes contribute protons (H+) or hydroxide ions (OH-) to these reactions, ultimately producing water and salt formation as a result. Acid-base reactions play an essential part of many chemical processes including neutralizing acids and bases.
- Electrochemical Processes: Electrolytes play an essential role in various electrochemical processes, such as battery and fuel cell batteries. Electrolytes allow electrons to move more freely across an electrode through the movement of ions within their electrolyte solution facilitating energy conversion and storage.
- Solubility and Precipitation: Electrolytes have an impactful influence on solubility and precipitation reactions, such as solubilization or precipitation reactions. Certain ions present can change the solubilization rates of other compounds, leading to precipitates forming.
- pH Regulation: Electrolytes play an essential role in maintaining the ideal pH levels of solutions. Both strong and weak acids as well as bases play an essential part in controlling the acidity or alkalinity of solutions and are vital in controlling their respective chemical systems.
- Industrial Applications: Electrolytes play an essential role in industrial processes. Electroplating involves depositing metal coating onto substrates using electrolytes and electrical current.
- Biological Systems: Electrolytes play an essential role in biological systems. From nerve impulses and muscle contractions to maintaining pH balance in body fluids and maintaining an ideal osmotic balance. Electrolytes like sodium, potassium, calcium, and chloride play an integral part in these physiological functions.
Electrolytes play an invaluable role in chemical reactions across a variety of fields, from chemical manufacturing and energy storage to environmental processes and biological functions. Acknowledging their behavior and properties is vital for designing effective processes that use electrolytes, as well as understanding underlying principles governing systems both natural and engineered environments.
Conductivity and Electrical Conductance of Strong Electrolytes
Strong electrolytes exhibit high electrical conductivity and conductance due to their nearly complete dissociation into ions when dissolved in a solvent. Conductivity and conductance are critical properties for strong electrolytes’ ability to conduct electricity within solutions; their conductivity directly correlates to having many freely moving ions that carry an electric charge and facilitate current flow.
Electrical Conductivity: Electric Conductivity (s) is the measure of a material’s ability to conduct electricity. It can be defined as the reciprocal of electrical resistivity (r), with units typically expressed in Siemens per meter (S/m) or millions per meter (mho/m). For materials such as strong electrolytes, electrical conductivity tends to be significantly high due to an abundance of ions within solutions which allow charged particles to move easily through solution channels.
When dissolving in water, strong electrolytes undergo complete dissociation into cations and anions that are highly mobile and capable of carrying electrical charge. When voltage is applied across this solution, these ions migrate towards opposingly charged electrodes to produce electrical current flow.
Strong electrolytes’ high electrical conductivity makes them efficient conductors of electricity, making them indispensable in many electrochemical processes and devices that rely on charging efficiently.
Electrical Conductance:
The conductance (G) of a material measures its ability to permit the passage of electric current when exposed to an applied voltage. It’s the reciprocal of resistance (R), measured either in Siemens (S) or mills (mho).
Strong electrolytes typically exhibit high electrical conductance due to their high electrical conductivity; their electrical conductance is determined by factors like the concentration of ions in solution as well as the cross-sectional area between electrodes.
The conductance of a solution can be represented as G = sx A/d Where: G is electrical conductance measured in S or mho and s = Electrical conductivity in terms of S or mho per milliliter per centimeter umplut A = Cross-sectional area of solution in meters2 unplug D is the distance between electrodes in millimeters
Strong electrolytes offer superior conductance for the efficient movement of ions and electrical charges in their solutions, making them essential components of many electrochemical applications and electrical systems.
Strong electrolytes possess excellent electrical conductivity and conductance properties that enable them to serve as effective conductors of electricity, making them useful in many different technological, industrial, and scientific applications.
Illustrative Chemical Equations for Strong Electrolyte Dissociation
Below are illustrative chemical equations illustrating the dissociation of strong electrolytes in water:
- Dissociation of Hydrochloric Acid (HCl): Whilst hydrogen chloride (H+) + chloride ions (Cl-) are present, dissociation will still occur and create hydrochloric acid in solution form.
- Hydrochloric acid (HCl): Dissolves completely into hydrogen ions (H+) and chloride ions (Cl-) when exposed to water, thus producing two distinct species of ions: hydrogen (H+) and chloride ions (Cl-).
- Dissociation of Sulfuric Acid (H2SO4): H2SO4(aq) = 2H+(aq) + SO42-(aq).
- Sulfuric acid (H2SO4: Dissociates into two hydrogen ions (2H+) and one sulfate ion (SO42-) when present in water solutions.
- Dissociation of Nitric Acid (HNO3): HNO3(aq) – H+(aq) + NO3-(aq)
- Dissociation of Sodium Hydroxide (NaOH): With increasing concentration, NaOH dissolves to Na+ and OH- in solution form.
- NaOH dissociates into sodium (Na+): Ions and hydroxide (OH-) ions in an aqueous solution, producing two distinct species.
- Dissociation of Potassium Hydroxide (KOH): KOH(aq) = K+(aq) + OH-(aq).
- Potassium hydroxide (KOH) dissociates: when exposed to water, yielding potassium ions (K+) and hydroxides ions (OH-).
- Dissociation of Sodium Chloride (NaCl): NACl(aq) = Na+ + Cl-
- Dissociation of Potassium Nitrate (KNO3): Washout process with K+(aq) + NO3-(aq).
- Potassium nitrate (KNO3): Dissociates into potassium ions (K+) and nitrate ions (NO3-) when dissolved in water, producing two distinct species of ions: potassium (K+) and nitrates (NO3-).
These examples demonstrate how strong electrolytes can dissociate into their constituent ions in an aqueous solution and, due to this reaction, form high concentrations of ions with increased electrical conductivity and conductance within the solution. Such reactions provide key insight into understanding their behavior during various chemical and electrochemical processes.
Conductivity and Electrical Conductance of Weak Electrolytes
Weak electrolytes tend to exhibit lower electrical conductivity and conductance than strong ones due to their partial dissociation into ions when dissolved in solvents like water. While weak electrolytes undergo dissociation, only some molecules actually convert into ions, leaving only a limited number of charged particles freely movable through the solution.
Electrical Conductivity:
Electric conductivity (s) is the ability of materials to conduct electricity. Weak electrolytes have lower electrical conductivities due to only dissociating into ions a small portion of molecules in solution; as a result, concentration levels drop limiting efficient transmission of charge through their solutions.
As an example, when dissolving weak acids like acetic acid (CH3COOH), only a portion of its molecules dissociate into hydrogen ions (H+) and acetate ions (CH3COO-), thus limiting how many available ions carry a charge and contribute to electrical conductivity; leading to lower conductivity than with strong acids like hydrochloric acid (HCl).
Electrical Conductance: (G) is a measure of how readily an object allows electrical current to pass through it when subjected to an applied voltage, influenced both by its electrical conductivity and by dimensions between electrodes.
Due to weak electrolytes’ lower electrical conductance and limited concentration of ions, their electrical conductance is reduced. Since dissociation is partial in these weak electrolytes, their number of free-moving ions decreases; hence resulting in reduced conductance.
Mathematically, the conductance of a solution may be expressed as follows: G = sx A/d for solutions that use S or mho as their measurement system: Where:
G is electrical conductance measured in S (or mho), while s is conductivity (in S or mho per mille). A is the cross-sectional area (m2) in which this solution exists while d represents distance between electrodes in millimeters (mm).
Weak electrolytes’ lower electrical conductivity and conductance is caused by their partial dissociation into ions. While weak electrolytes still conduct electricity to some degree, their conductance properties are less efficient than strong ones. Yet despite having limited ionization properties they remain essential components in chemical and biological systems and play important roles in maintaining pH balance, buffering solutions, as well as in influencing solubility reactions that lead to precipitation reactions.
Illustrative Chemical Equations for Weak Electrolyte Dissociation and Equilibrium
Below are illustrative chemical equations for the dissociation and equilibrium of weak electrolytes in water:
Dissociation of Acetic Acid (CH3COOH):
CH3COOH(aq) = CH3COO-(aq) + H+(aq).
Acetic acid (CH3COOH) is a weak electrolyte that, when dissolved in water, dissociates into acetate ions (CH3COO-) and hydrogen ions (H+). The reaction remains in an equilibrium state, with some molecules of acetic acid remaining undissociated.
Dissociation of Ammonia (NH3): When combined with H2O(l), ammonia forms NH4++OH- (both as inorganic ions).
Ammonia (NH3) is a weak base that, when mixed with water, forms ammonium ions (NH4+) and hydroxide ions (OH-) through an equilibrium reaction. Both forward and reverse reactions take place simultaneously, leading to partial dissociation of ammonia molecules.
Equilibrium for Carbonic Acid (H2CO3): H2CO3(aq) = HCO3-(aq) + H+(aq).
Carbonic acid (H2CO3) is a weak electrolyte that undergoes an equilibrium reaction when dissolved in water, producing bicarbonate ions (HCO3-) and hydrogen ions (H+) as products of dissociation. An equilibrium exists between its undissociated form and dissociation products.
Dissociation of Hydrogen Sulfide (H2S): H2S(aq) = H+ + HS-
Hydrogen Sulfide (H2S) is a weak electrolyte that partially dissociates into hydrosulfide ions (HS-) and hydrogen ions (H+) when exposed to water, creating an equilibrium reaction between undissociated H2S and its dissociation products.
Dissociation of Acetic Acid (CH3COOH) in Water with water acting as a base:
CH3COOH(aq) + H2O(l) = CH3COO-(aq) + H3O+(aq).
This equation illustrates the reaction between acetic acid (CH3COOH) and water acting as a base, leading to the formation of acetate ions (CH3COO-) and hydronium ions (H3O+), with hydronium being formed via proton transfer from acetic acid into water.
These chemical equations illustrate the partial dissociation and equilibrium of weak electrolytes in water. Unlike strong electrolytes, weak ones do not fully dissociate, leading to equilibrium between undissociated molecules and their dissociation products – leading to lower concentration of ions and electrical conductivity as a result of lower electrical conductivity compared with strong ones. Still, weak electrolytes play essential roles in various chemical and biological systems, contributing to pH regulation, buffering processes, or other crucial functions.
Examples in Everyday Life and Industrial Applications
Electrolytes play an essential role in both everyday life and industrial applications, providing energy to cells. Below are examples of strong and weak electrolytes:
Everyday Life Examples:
Strong Electrolytes:
- Table Salt (NaCl): Table salt is an effective electrolyte, completely dissociating into sodium ions (Na+) and chloride ions (Cl-) when dissolved in water. This makes saltwater an excellent conductor of electricity which has many applications across various fields of application.
- Battery Acid (H2SO4): Battery acid is a strong electrolyte used in lead-acid batteries to facilitate electrical current flow inside of them and store and release energy during charging and discharging cycles.
- Vinegar (Acetic Acid – CH3COOH): Vinegar is an example of a weak electrolyte as its chemical constituents partially dissociate into acetate ions (CH3COO-) and hydrogen ions (H+). Due to its mild acidity properties, vinegar makes an ideal product for many culinary and cleaning uses.
- Ammonia Solution (NH3): Ammonia is a weak base that partially dissociates into ammonium ions (NH4+) and hydroxide ions (OH-) when dissolved in water, providing cleaning products with ammonia-based cleaners use its weak basic properties for effective cleaning purposes.
Industial Applications:
Strong Electrolytes for Electroplating:
- Electroplating processes: Use strong electrolytes with metal ions such as Cu2+ or Ni2+ to deposit layers of metal onto conductive substrates. Strong electrolytes enable efficient ionization and deposition processes, creating uniform coverage of metal coating.
- Electrolysis: Electrolysis uses electrical current to drive chemical reactions that would not occur spontaneously. Strong electrolytes play a crucial role in electrolysis processes as they facilitate ion movement and cause desired chemical transformations.
- Weak Electrolytes: pH Regulation and Buffering: Weak electrolytes such as acetic acid (CH3COOH) and ammonia (NH3) act as buffers to regulate and stabilize the pH levels in solutions, accepting or releasing protons according to system needs to balance out acidity or alkalinity fluctuations and stabilize solution characteristics such as acidity or alkalinity.
- Pharmaceutical Industry: Within the pharmaceutical industry, weak electrolytes are often utilized as ingredients in drug formulations to control drug release rates and maximize effectiveness within the body.
Different substances exhibit differing degrees of electrolytic behavior, with concentration and temperature playing key roles in classifying strong or weak electrolytes. Understanding their behavior is integral for optimizing chemical processes, industrial applications and technological innovations.
Practical Importance of Understanding Electrolyte Strength
Understanding electrolyte strength is of great practical significance in various scientific, industrial, and everyday applications. Some key implications include:
- Electrochemical Processes: Electrolyte strength is of great significance in electrochemical processes like batteries, fuel cells and electroplating. Ionization efficiency plays a significant role in efficient charge transfer and energy conversion between these systems; knowing how different electrolytes perform can help optimize performance and prolong lifespan of electrochemical devices.
- Water Treatment and pH Regulation: When it comes to water treatment, understanding strong and weak electrolytes is key to regulating its pH to desired levels. Strong acids and bases neutralize water while weak electrolytes like buffers provide stability by helping maintain stable pH levels throughout various chemical processes.
- Medical and Biological Applications: Electrolyte balance is vital to the proper functioning of biological systems, including our bodies. Understanding how strong and weak electrolytes behave within biological fluids is critical in diagnosing and treating medical conditions related to acid-base imbalances or electrolyte disorders.
- Industrial Chemistry: Electrolyte strength knowledge is integral in designing efficient chemical processes and optimizing reaction conditions, and selecting appropriate electrolytes can significantly impact reaction rates, product yields, and overall process efficiency.
- Corrosion and Coating Technologies: Understanding electrolytic behavior is critical in mitigating corrosion in metal structures. Electrolyte solutions can affect corrosion rates and effects; coatings may also provide additional protection from further corrosion damage.
- Energy Storage: Electrolytes play an essential part in energy storage technologies such as supercapacitors and redox flow batteries, making understanding their strength critical in designing high-performing energy storage systems.
- Environmental Remediation: Electrolysis and other electrochemical processes using electrolytes are used in environmental remediation to treat polluted water and soil, optimizing treatment processes and efficiently eliminating pollutants. Knowledge of electrolyte strength helps optimize treatment processes and remove pollutants more effectively.
- Chemical Analysis: Electrolytes are widely employed in various analytical techniques, such as ion chromatography and capillary electrophoresis, to separate and quantify ions present in samples. Understanding electrolyte strength is paramount in order to produce accurate analytical results.
Summary
Electrolytes with high strength fully dissolve into ions after being dissolved in water. This results in an extremely high conductivity. Examples include sodium chloride as well as hydrochloric acid. Electrolytes that are weak dissociate only in part which results in less ions as well as less conductivity. Ammonia and Acetic Acid are two such examples. The main difference is in the amount of ionization, and consequently the capacity to transmit electric current.